ENSPIRING.ai: Beyond Planets - The Quest for Exomoons
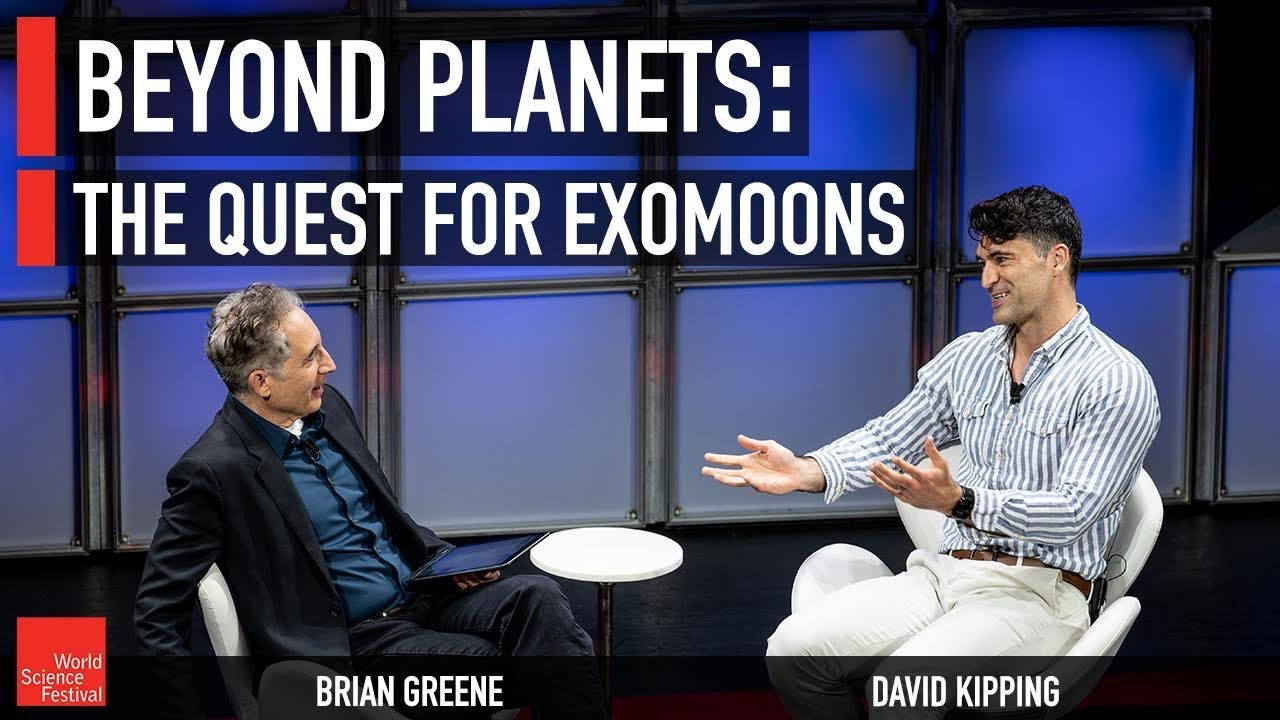
The video explores the significant advancements in the study of exoplanets and exomoons. It highlights the confirmation of thousands of exoplanets outside our solar system, and ongoing efforts to study their temperatures, atmospheres, and potential for life. The discussion extends to the niche field of exomoons, which might hold vital clues in understanding extraterrestrial life. The video introduces David Kipping, an astronomer who has dedicated significant research toward discovering exomoons.
The video presents a historical overview of how exoplanets were discovered, from early speculative claims to confirmed discoveries in the 1990s using precise astronomical techniques. It explains the process of detecting exoplanets through methods such as transit observation and radial velocity. The narrative emphasizes how the field evolved from skepticism to a central focus in modern astronomy, marked by collaborations with missions like Kepler and the James Webb Space Telescope.
Main takeaways from the video:
Please remember to turn on the CC button to view the subtitles.
Key Vocabularies and Common Phrases:
1. exoplanet [ˈɛksoʊˌplænɪt] - (noun) - A planet that orbits a star outside our solar system. - Synonyms: (extrasolar planet)
The confirmation of exoplanets with the total number
2. astrometry [æˈstrɒmɪtri] - (noun) - The branch of astronomy that involves precise measurements of the positions and movements of stars and other celestial bodies. - Synonyms: (celestial mechanics, astrophysics)
Because Friedrich Bessel had been discovering binary star systems using astrometry.
3. pulsar [ˈpʌlsɑːr] - (noun) - A highly magnetized rotating neutron star or white dwarf that emits beams of electromagnetic radiation. - Synonyms: (neutron star, radio star)
Essentially, it was a similar approach in terms of looking for wobbling stars. But the pulsar enables you incredible precision because it is this lighthouse.
4. resonance [ˈrɛzənəns] - (noun) - The reinforcement or prolongation of sound by reflection or by the synchronous vibration of a neighboring object. - Synonyms: (harmony, synchronization, reverberation)
So some of these systems, they get trapped in resonances with each other, which means, imagine that the earth had a companion planet that happened to have a, say, a two year orbital period
5. astrobiologists [ˌæstroʊbaɪˈɒlədʒɪsts] - (noun) - Scientists who study the origin, evolution, distribution, and future of life in the universe. - Synonyms: (life scientists, space biologists)
So it's thought that having especially astrobiologists, I often say that one of the criteria potentially, for certainly complex life might be one of these magnetospheres.
6. magnetosphere [mæɡˈniːtəˌsfɪr] - (noun) - The region around a planet dominated by the planet's magnetic field, protecting it from solar radiation. - Synonyms: (magnetic shield, radiation barrier)
So can you tell us what we're seeing here? Yeah, this is a simulation of the earth's magnetosphere, which protects us from cosmic radiation, especially solar radiation
7. doppler shift [ˈdɑːplər ʃɪft] - (noun) - The change in frequency or wavelength of a wave in relation to an observer who is moving relative to the wave source. - Synonyms: (frequency shift, spectral shift)
And as these beams sweep by, you can, like clockwork, they should happen. But if there is something around, it will cause a doppler shift.
8. eclipse [ɪˈklɪps] - (noun) - An obscuration of light occurring when one celestial body moves into the shadow of another. - Synonyms: (obscuration, transit)
This is not a total eclipse, but a partial eclipse.
9. rogue planets [roʊɡ ˈplænɪts] - (noun) - Planets that do not orbit any star and instead drift through space. - Synonyms: (interstellar planets, wandering planets)
And then there's rogue planets, planets which get kicked out.
10. exomoons [ˈɛksoʊmuːnz] - (noun) - Moons that orbit exoplanets, planets outside our solar system. - Synonyms: (extra-solar moons, moons of exoplanets)
Now, what one hears less about is the search for exomoons, moons orbiting exoplanets.
Beyond Planets - The Quest for Exomoons
One of the other great success stories of the modern age, really a vital success story of recent years has been the confirmation of the existence of planetary systems outside of our own solar system. The confirmation of exoplanets with the total number. Now, I don't know the exact value, but it's certainly well into the thousands. And much work is now underway to determine the detailed nature of many of these exoplanets. We want to figure out their temperatures, whether they have atmospheres, and if they do have atmospheres, what's the composition, and so on. As all of this is a vital step toward understanding how special or not the conditions that, at least on our planet, were conducive to the formation of life.
Now, what one hears less about is the search for exomoons, moons orbiting exoplanets. I mean, here in our solar system, right. We have known for hundreds of years that moons are really prevalent. But the question is, is this the case for other systems of planets orbiting other stars? And as we will see, this may be a vital question in the ongoing search for extraterrestrial life, as our guest in this conversation will help us to understand, as he has been spending many years trying to answer the question of whether there are actually exo moons.
So I am pleased to introduce David Kipping, who is a professor of astronomy at Columbia University, where his lab is primarily focused on discovering new planets and moons. You may know him from previous appearances on how the universe works in Star Talk, and he also runs his own YouTube channel called Cool Worlds. So thank you. Welcome. Great to see you. So, exomoons. Yes. Is there a huge community of people looking for exomoons? Believe it or not, it's a fairly niche topic. There are probably a few dozen of us, I would say, in the world who are interested in this, and hundreds, if not thousands of scientists who are studying exoplanets. We see this as the new frontier. We think once we get them, everybody's going to be on the bandwagon kind of thing.
Yeah. So toward that end, and just to sort of place everything in context, I thought it might be good to go through some of the timeline of exoplanets and then head into exomoons as a natural outgrowth and as you say, perhaps the next frontier. So in thinking about the history of exoplanets, where is a good place to pick it up? And please, maybe you'll begin with where we have in our graphic, because otherwise it'll be really awkward.
Yeah, this is. This is Captain William S. Jacob. Captain. That was his title. Not a not doctor. And he was at Madras Observatory in India and captain of. He was in the navy for a while. He was in the navy, yeah. And so he ended up at this back end observatory in India. And he was inspired, I think, at the time, because Friedrich Bessel had been discovering binary star systems using astrometry. So astrometry means a wobbling star. So you see a star and you keep measuring its position, and it's not in the same place. It's changing in a periodic way. And so he was probably inspired by that and thought, maybe I could do the same thing to find planets. How could they do measurements that precisely? I mean, it sounds hard.
I mean, to someone who only deals with equations, I stand in awe of anything that you guys are able to do with your hands or with telescopes. It's unbelievable. And in fact, you know, he clearly wasn't as accurate as he thought he was because the planet that he claimed was spurious. So this was a system called 70 of Fuchi. He thought this binary star system had some excess wobble in there. And perhaps, you know, this isn't that strange, because around the same sort of time we discovered or claimed to discover Neptune that way. Yes, for sure. He looked at Uranus's motion. He saw that wobbling. So there was a lot of astronomers who were thinking, hey, we can do this. We can find extra objects this way. But it was all essentially by eye. You were looking through the telescope, noting down the position of Ra and deck of where you thought it was. And this was pre having computers to help us automatically measure those positions. So amazing. It really is amazing.
And then I think sort of the natural next step is sometime later, but in between, long time later, but in between, there was a lot of claims. Oh, continuously. And it brought a lot of disrepute to the idea of even looking for exoplanets. You know, these days, there's kind of a giggle factor when we talk about looking for alien life. A lot of people even today, still kind of snigger at it. Back then, it was exoplanets, including one of our colleagues, who.
Well, anyway, that's a. That's a different story. Different story. Yes, keep on going. Even so, during this period, there were many, many claims. Barnard Starr was a very famous example in 1960s. And so a lot of astronomers kind of thought the idea of looking for exoplanets was very fringe science and wasn't to be taken too seriously. And so it took an enormous step forward in precision before it was undeniable, which is what we had in 1992 with the pulsar planets.
And so what made their work stand out? I mean, what was different about the approach? Essentially, it was a similar approach in terms of looking for wobbling stars. But the pulsar enables you incredible precision because it is this lighthouse, this tiny mechanism built in. You see these beams flashing by. You come at the north and the south pole of the pulsar. And as these beams sweep by, you can, like clockwork, they should happen. But if there is something around, it will cause a doppler shift, like the siren of an ambulance going by the pitch change. And so using these clocks, these astronomical clocks, which are extremely precise, they were able to pull out this signal.
That was enormous significance with, you know, typically in astronomy, a new discovery is what we call a two or three sigma detection. The Higgs boson, when it was found, was a five sigma detection. This was, like, over 2025 sigma. It was just so clear. You could see it by eye. Nobody could challenge. This was a real signal they were seeing. And then next milestone moment is just a few years later.
Yes. And so this was important because even though the pusaw was an incredible discovery, we have now planets around pulsars, the skepticism persisted because this was now 150 years of false alarms. And so some people wondered, maybe there's something astrophysically going on with these pulsars we don't understand, something intrinsically that was causing this small change. It could be a glitch or something.
And after all, a pulsar, if you think about it, is an extraordinarily strange place to expect a planet, for that is a star which has gone supernova in its past. That's the only way to make a pulsar. And these planets were parked fairly close to the star, so why were destroyed or something like that? This doesn't make any sense. And it's still kind of a puzzle as to what they're doing there. What Michel mayor and Delia Kellers did was to found a planet around a sun like star. And so once we found that this was a big step forward, a lot of people said, well, now it's harder to question this. There's no reason why sunlight. Stars, of course, should not have planets. Right? And then, of course, the big moment in this temporal progression is the transits. Transits and then the Kepler launch.
Yeah. So, Dave Charbonneau and Tim Brown led the first discovery of HD 209 458 b. We have these wonderful names for exoplanets. They're like license plate numbers. I apologize. We have such terrible names. Astronomers are good at many things, but naming planets is not one of our highlights.
And what was amazing about this was at this point, there'd been about ten planets discovered using the same method that Michel Mayo and Didier Kellogg used. We call this the radial velocity method. It's a wobbling star method. You look at the star, you see it coming towards you, getting blue shifted, coming away from you, getting red shifted. So they use that method to discover about a border of ten to 20 planets. And one of those. These planets were all very strange, by the way. They were very close to the star. We call them hot jupiters now. So things which people, again, were still skeptical of because they were thinking, again, maybe the star is doing something we don't understand. How could you have a Jupiter sized planet so close? I'm talking 20 times closer than Mercury's orbit around the sun. People just didn't understand how that was possible. And so it was predicted that just geometrically, if this population is out there, the probability of getting an eclipse is about 10% per hot Jupiter.
So they said, look, we've got ten or 20 of these things. Show me the money. One or two of these should be eclipsing if your theory is correct, and they were the first ones to go out, chase it, and really get that proof of the pudding that it eclipsed, just like the total eclipse we had in the US, not recently, but this is not a total eclipse, but a partial eclipse. And they saw that eclipse and it proved, this is it. And now I think at that point, everyone was like, these exec plants, they must be real.
And is that helpful in getting ultimate funding for serious missions that would no longer just be trying to establish that there are exoplanets, but start to find them and catalog them and study them? For sure. For sure. We've gone from this period of, as I said, like, from 150 years, pretty much of everyone thinking this was a fringe science, not real, legitimate stuff, to now having missions like the Kepler mission, which launched in 2009. If you look at the James Webb space telescope, its allocation of telescope time, about a third of its telescope time, is dedicated to exoplanets, despite the fact that telescope was not designed to do exoplanets, it was designed to do cosmology experiments, of course. Yeah. And yet we have about the same chunk of telescope time now as cosmologists do, which I'm sure they're not happy about. But we are very pleased with that situation. We're all one. We're all one now.
So as of today, then, I don't know the exact number, maybe you do. Or rough order magnitude. How many exoplanets have we found? It's in excess of 5000. It's changing every day, so, you know, every day excess of 5000, yes, confirmed exoplanets. So we're expecting this number to balloon in the coming years. It's expected. There's a new telescope called Roman coming online in the coming probably three or four years. And this telescope should be able to deliver a water of hundreds of thousands of exoplanets. This is not going to stop. And of course, there's about 100 billion stars in the Milky Way. So we are at the very tip of the iceberg of our galactic exploration.
And so is the expectation then that it's the norm for a star to have planets? It certainly appears that way. I think on average there is more than one planet per star in the sky. But of course, not every star has a planet, but it seems that the majority do, typically stars like the sun. It's very, very common. You look at a star that's much more massive than the sun, that doesn't live as long. It's like the James Deans, like, they live fast, die young, these stars. They probably don't have time to get plants, but anything that's similar to the sun, we see plants everywhere we look. And so my guess is that they are ubiquitous across there.
So we're talking, even in our galaxy, in hundreds of billions of planets, potentially, I mean, maybe even trillions. I guess if it's like a factor of two or three, it could well be. And then there's rogue planets, planets which get kicked out. There may be, very well more rogue planets, free floating planets between the stars than there are stars themselves. A lot of simulations show that there's hints in the data this might be true. So it's fascinating. So lonely now.
So I'd like to just drill down a little bit on how you go about establishing the existence of an exoplanet. And there's this idea of watching the planet as it transits across its host star. And I guess just looking at the light output and seeing how much of it is occulted by the presence of the planet. Is that the essential idea? It's a beautifully simple experiment. You essentially just take a photograph of a star. Typically with Kepler, it was every 30 minutes it would take a photograph of a star, and you just measure, even though you can't see the planet in the star, because, of course, as fantastic as these telescopes are, we cannot resolve what you see on the screen right here, you cannot resolve a planet star like this, but what you can do is very precisely measure how bright is that star in each one of those photographs. You line up these photos and you just make these plots of how bright was the star versus time. And lo and behold, you see these dips, and that's our first telltale sign that we have a planet. It could still, at that point, be something else, maybe a binary star system. That's when the investigation work begins. But that's the first real clue that we have a planet.
And, in fact, you can even do this with the possibility of multiple occlusions. I guess there's another version of the same. Yeah. And there are some wonderful dynamics that go on with these multi planet systems. So some of these systems, they get trapped in resonances with each other, which means, imagine that the earth had a companion planet that happened to have a, say, a two year orbital period. Mars is not quite at that orbital resonance, but then there'd been a two to one resonance with each other. And in a two to one resonance, it's like pinging the glass and it kind of smashing. They amplify each other's interactions, and they can have enormous wobbles in space. So one of the projects in my team right now is to look at these wobbles. We see thousands of these planets which undergo these wobbles. And that tells us, just like with Uranus and its discovery of Neptune, there must be thousands more planets further out in these systems yet to be revealed. And to detect the wobble. Is it a similar transit type of observation? Yes.
We essentially just time, we take that dip in light and we very precisely write down the time at which it happened. And really, when you think about the earth, you'd expect New Year's Day to happen January 1 every time. But what we see is that sometimes New Year's Day happens two or three days early, essentially, is what we're seeing. So it sort of got pulled this way or pulled that way by some companion planet.
So what would you say the discovery of these 5000 plus exoplanets has taught us, just sort of on a 30,000 foot level? I mean, is our solar system run of the mill? You know, is it just us out there many times over in some sense, or is it very different out there? That is the question, isn't it? I think it's still a question we're wrestling with at the 30,000 foot level. We know that planets are very common. We know that there are many different types of planets than that which we expected. For instance, there is no planet in the solar system which is in between the size of Neptune and the earth. So Neptune is about four times the diameter of the earth. But there are many, many planets that are two to three times the size of the earth. In fact, this is the most common type of planet we have discovered in the universe. We call these mini Neptunes, but maybe they're actually super Earths or mega Earths, like scaled up rocky things or scaled down gaseous things. We still are not sure exactly what those objects are, but they're everywhere. Seeing the data, and we noticed that the architectures of these systems do not often resemble the solar system.
There are some, but I would say the solar system is a rare instantiation. Something like 10% of the architectures we see look consistent with that of the solar system. Besides that, you get these highly eccentric planets, which are more like comets sweeping around. You get these hot Jupiter systems. You get systems of these compact mini Neptunes, like six or seven of them, all patched within the orbit of Venus. So there's enormous diversity. And 10%, though, is still huge on the astronomical scale of things.
Yeah, it is a lot. But remember, sunlike stars are themselves a rare breed. Only about 10% of stars in the universe are truly sun like, as in g type star, yellow dwarf stars. Then of those, maybe you have about 10% that have a Jupiter. That's the statistic I'm really thinking of there. How many have a true Jupiter analogous? That's about 10%. Beyond that, how many of those have true earths as well? We actually don't know the answer to that. This is called eta Earth, the fraction of sunlight stars that have Earth like planets.
Astronomers call it eta Earth. Iter is like a greek symbol, so it's like the fraction number, and that is a number that Kepler was promised to deliver us. And it turned out the stars were just a little bit noisier and trickier than we imagined they would be, and it prevented us from finding a catalog of true Earth. And what I mean is that, again, sun like stars, we have Earth sized planets at the right temperature, potentially for life around smaller stars. But around yellow dwarfs, they have been elusive, and that remains an enormous challenge for us. Yeah, for sure.
So let's now turn to the idea of exomoons, which is. So this is obviously a wonderful success to be able to find all these exoplanets. What's the motivation? Is it just that you want to further know whether the solar system is kind of a dime a dozen, where a dime is the 10% in monetary terms. Did you see that move right there? Yep. Or whether moons are highly special. Is it just sort of an abstract itch that you want to scratch, or is there real motivation to knowing about moonshi I mean, for me personally, when I started this, it was more like, why climb Everest? Because it's their type mentality.
I think physicists often draw to those kind of challenges of like, let's see if we can do this, if we can pull this off. But there are real, extremely important physical reasons to do this science. The first one is if you've only seen a Sci-Fi film like Avatar or Star wars, you know, they often play with the idea of a habitable moon. So it may very well be. I mean, we already know there are more gaseous planets in the habitable zone than there appear to be rocky planets. So if some fraction of those gaseous planets have themselves a moon system, there may very well be more habitable moons than the rock planets in the universe, which is a staggering thought. We'd be the freaks, right? The aliens would be looking at us thinking, that's kind of weird. They live on a planet like, what? What must that be like? I stay awake at night thinking about that sometimes. The other reason is that moons may actually help planets be habitable. If we find an Earth twin, one of the first questions we might ask is, does it have a moon twin? Because our own moon seems to have had an enormous influence on our own evolution.
It actually stabilizes the axial tilt of the earth. So we have an actual tilt of about 23 degrees. That's what gives us the seasons summer and winter. And if it was not for our moon, it's likely that Jupiter's influence would essentially destabilize it, and it would end up drifting and wandering over millions of years, which would be probably fine for life, but not so good for agriculture and civilization as we know it. But isn't the moon's existence tied to the tilt of the earth? I think many have thought that the formation of the moon through a collision with this planet like object, Mars sized theia, I guess people have called it, that may have resulted in the tilt of the Earth.
In fact, we have some animation. Maybe you could take us through on the potential formation of our moon. Yeah. So we have the splash here of a Mars sized planet, essentially, that's wandering through the solar system. This is happening sort of four and a half billion years ago. We were rewinding the clock. It smashes into the Earth. The Earth originally would have been a little bit larger than it was today, maybe 50% more massive or something. It smashes into it. And actually, in most of these simulations, it's kind of hard to see. The original impactor is completely vaporized, essentially annihilated.
And the thing which forms the moons, in this case, it actually looks like there's two moons which are forming, is not really the impact, but actually a portion of the earth itself is ripped off and recoalescence. And that kind of explains why, when the Apollo astronauts went to the moon and they brought back moon rocks, and we looked at what's called the isotope ratio of those rocks, it was identical to that of earth rocks, which was a puzzle because that doesn't happen for any of the rocks in the solar system. So it's really implied that the earth and the moon formed from the same stuff, which somehow got mixed together. So this makes a nice explanation. There's still many challenges. We still don't fully understand exactly what happened here. It looks like you're forming two moons, which does often happen in these simulations, and often these two moons eventually coalesce in some way.
And so when you're looking for exomoons, and we'll look at, toward the end, a candidate, I think, that you have from your data, are you envisioning that it's this very process analogous to this which has happened? It may be this process forms a moon which is exceptionally close to the planet. So if you were born, if you were around on the earth at this time, it would have been quite staggering. You would have looked up at the sky and seen the first moonrise, and it would have filled a huge fraction of your sky. It would have been a breathtaking view over time because of tides. The tides that we have, that is an energy dissipation process driven by the moon, and that's causing the moon to migrate away. And it's still migrating. It's still. It's about an inch. We're not gonna have total solar eclipses in like 600 million years or something like that. I probably won't be around, so I'm not too worried about that, but probably eventually that'll stop.
And so this is one way. This is one way we're interested in. But there are other methods. There could be a capture. A planet could, instead of smashing. I think we have a version of that. Yeah, this is. This is an idea of, in this case, it looks like a tidal capture. So we talked about these tidal forces that lead to dissipation in the Earth moon system. And you've probably heard of the idea of spaghettification for a black hole. Like if you fell into a black hole, the tidal forces would be so strong that they essentially pull you harder at your feet and less at your head. And this would dissipate energy.
Again, the object. If you want to capture a moon, the great challenge in the solar system is that everything's moving very fast. So if you want to capture something, you have to break it. You have to apply some kind of break to get it below the escape velocity of the planet. If you can apply a break, you can capture it. And so a lot of the theoretical challenge is how could an object suddenly put on the brakes? And one idea is the tidal forces could be strong enough for these close encounters to do that. The atmosphere might even play a role in.
So you'd have to get pretty close to the planet in that these have to be very close together, probably the most. And people have simulated that. And really the atmosphere. The atmosphere, I'm not so sure about. The tidal method certainly has been explored.
Um, probably the most popular idea is what's called a binary exchange mechanism, which is a little bit conceptually trickier to look at. But this is actually how we think Triton formed around Neptune. So imagine you have two objects, two proto moons, and they're orbiting each other. There's many of these in the outer Kuiper belt of the solar system, like an asteroid belt.
And so as they wander towards the planet, let's say you're the planet over here, and you're. You're moving through space at a certain point in time. Let's say you're going towards the audience here at this point in time. This object is now moving at the same kind of speed as you, in the same direction. So your relative velocity is now zero. And so that allows the capture to happen below the escape velocity.
This object, in the other hand, is going the complete opposite direction. So it gets ejected from the solar system altogether and becomes one of these potentially rogue planets. We think that's probably how Triton formed. I see. Wow. And the issue of life on these other moons is one that you already made reference to, but I wonder if you could just flesh that out a little bit.
Now, there is this notion that there can be a kind of protection mechanism that's going on. So can you tell us what we're seeing here? Yeah, this is a simulation of the earth's magnetosphere, which protects us from cosmic radiation, especially solar radiation. And so it's thought that having especially astrobiologists, I often say that one of the criteria potentially, for certainly complex life might be one of these magnetospheres, but that requires that you have a planet which has a sufficiently large mass and therefore core that it can sustain one of these dynamo processes that generates the magnetic fields. So one of the concerns is what happens if you have something like Mars or the moon, which is too small to generate a significant magnetic field like this? It would therefore be bombarded with radiation.
Surface life wouldn't be possible. We'd be in trouble. But the kung fu move, the ninja move that moons can have here is that they may be too small to have their own magnetic field. They'll just hide behind. They can borrow, they can use for some fraction of the time, not the whole time, but maybe 30, 40% of the time, they can be inside this veil of the magnetic magnetopause of the planet. And that can give them sufficient protection. Maybe you can imagine life coming out during those regions, during those times, and then hide underground subterraneously or something the rest of the time.
When you think about life on moons, are you solely thinking of moons around exoplanets, or what about moons around planets in our own solar system? Where do we stand on that? Yeah, I mean, moons in their own solar system are fascinating. There's obviously a lot of interest in Europa, Enceladus as potential seats for life. We have missions coming up that will not be landing on the surface of these objects just yet, like the Europa clipper and juice that will be giving us high resolution images of the surface. Is this Europa I think we're looking at here? Yes.
And so Europa's fascinating because when you look at its surface, if you compare it to the moon, if you think about the moon, it's kind of a boring place. It's just craters. That's all you have. There are not very many craters on Europa. And what that tells us is that there must be some resurfacing process. This surface is not 4 billion years old. It's a fresh surface. The obvious explanation as to what might be going on is that there is, you know, because it's an icy surface. You can see that just from the imaging that perhaps this ice is melting periodically.
So the heat source that's driving it. Yeah. The heat source in this case can't be the sun because it's really too far away from the sun. So the heat source has to be internal. We think the most likely culprit is, again, I'm going to say tides, but it's going to be coming from the fact that it's in this resonant chain with its companion moonshi. And these resonances keep pushing Europa. Europa wants to get into a circular orbit. Everything kind of wants to go into circular orbit because of tides. But the other moons which are in this resonance state, the two to one, the three to one state, they keep stretching it unwillingly into an elliptical orbit.
And whenever you have something in elliptical orbit, it's sometimes closer to the gravitational potential and sometimes further away. And that causes these tidal forces to mush it in and out, in and out, in and out. And so that may be generating a heat source. We certainly see it with IO. When you look at IO, the innermost moon, it's splattered with volcanoes. We know for certain it is happening there. And we see lots of evidence to support this is also happening inside Europa. And so there's almost certainly an ocean beneath there.
And that's extremely exciting for the prospects of life, of course. And this potentially is the same kind of physics that would have, be governing exo moons in some extra planetary system. Right? So this is not necessarily something special to our solar system. And what's really fun is that this process can play out without a star. We talked about these rogue planets, these planets which get ejected out of their solar system. I think they're called, astronomer called them Wolfenstein worlds. I don't know quite why he named them that, but there's an idea that you could have these rogue planets that have moons, and those moons could be tidally heated and sustain biospheres.
All life then would be under surfers. Yes. And so there could truly be. We could truly be very strange then. In that case, we're like the only surface dwelling life. Yeah. And everyone would just be underneath the surface. So they'd come here for, like, the Florida vacation or something. Yeah, yeah. They're all whales and dolphins out there. Just the strange things with fingers.
All right, so now, sort of to the. To the main question then, after all this motivation, interest for exomoons, extraterrestrial life, and so forth, where do we stand on potential exomoon candidates? I've spent most of my career trying to push this field and to truly get these detections. So it has been a personal journey. And over the last, I'd say, 1213 years of trying to do this. Since my PhD, we've uncovered in my team two exomoon candidates, which I think are really the only two serious candidates. This is an example of one of these transits.
So the planet comes in and you see it block out some starlight but there's the moon. You see an extra dip on the right there. Correct. So you've got this little extra dip. This is real data that we captured with the Hubble space telescope of an exoplanet called Kepler 1625 b. I'm sorry again for the horrible names we have in astronomy. And this little object here we call.
I guess the question is, what do we call the moons? We have this system where we call the star the name of the telescope Kepler. And then the number is like, how many planets have you found so far? So this point, it was 1625th planet that was discovered. The b tells us the order of the planet. B is the first planet discovered. A is the star. So that's why we started b. It's kind of confusing. And then we get BCD for all the others. And then the question is, what do we call the moon? Should it be a roman numeral? Should it be a dash?
People argue about that. I tend to think it's like a rose by any of the names. Still smells as sweet. Let the pedants argue about exactly how to call it. But we do have this evidence. It is a very strange moon, if it's real. This is a super Jupiter exoplanet. So it's several times the massive Jupiter in an orbit similar to the earth around the sun. So it's pretty cool by exoplanet standards.
And the moon here is so large, it would be comparable to the size of Neptune if it was real. So this is, as you can see, this is like what we call about a five sigma detection that you're looking at. So sometimes the difference between a planet, a planet and a moon is really context. It starts to blur. It starts. I mean, is this a binary planet? Is this a moon? It becomes up for debate at that point as to how you really classify these things.
And so you don't consider this, though, to be proof of x. It's not enough for me personally. I would like to see this signal repeat. We would like to capture another one of these beautiful events. And the power of the exomoon hypothesis is that the moon will not be in that same place every time. So here, you see, it's over on one side. But we can now predict the orbit. And so we can predict, okay, in the next transit, it'll be over on the left. So we have a testable hypothesis, a verifiable, falsifiable hypothesis that we can go out, collect telescope data. And if we don't see it there, we can say we're done. This must have been an instrument glitch or the star did something funky at that time. We want to see that a second.
So when. When was this data? This was 2018. This data was collected, so 2017. So, like, what are you waiting for? We've tried. Trust me, we've tried. As I said at the beginning, a good place to start this whole conversation is that us exomoonologists, if you want to call us that, are a niche breed. There are not very many of us. And so when we write these telescope proposals, and we did, we said, hey, we should get another one of these transit. Unfortunately, the downside of having so many exoplanet, brilliant colleagues is that there's lots of other ideas. So you're really just waiting in line. We couldn't get the time for this one.
We do have telescope time in October coming up with a James Webb space telescope for a completely different planet. So not to check on this one. This one, because it's. I think a lot of people have a little bit of a knee jerk reaction to this one because it's so strange. It's a Neptune moon. Neptune, as my student used to call it. This is a strange object. And some people, like the first hot Jupiters, didn't believe them. Hot Jupiter? How can you have a hot Jupiter? That doesn't make any sense.
Means Jupiter so close to its star. Yeah. People just doubt the physicality of that, and people are doubting the physicality of this object. I see. We've had trouble persuading the community about that. I would just like to get the data and see. Either way, we've had an easier time with our next proposal, which was accepted with James Webb, which is going after a. The closest planet to Jupiter that we know of. So it is an exoplanet that has the same temperature as Jupiter, same mass as Jupiter, same orbit radius.
Everything is pretty much identical to Jupiter, and so it really should have the same moons that Jupiter has, unless something's massively wrong with our understanding of how planet formation works. And James Webb is the first machine humanity has ever built that could detect moons as small as the moons we have in the solar system. Kepler couldn't do that. Even Hubble cannot do that. James Webb is the first telescope which is sensitive enough to Ganymede, to IO, to Europa. And so that's why we're so excited about these observations.
If we can prove these more familiar moons exist, we'll probably get everyone a bit more comfortable with the idea that these things are really out there. And you say that's this coming October, and how long does it take? I mean, is it the kind of thing where you put it into the model computer and it rapidly tells you whether or not you found it? We hope so. We're preparing this summer with, with simulated data. We're getting everything ready. We want to get at that point where we can just push the button.
This data set is going to be extraordinary. It's 60, 70 hours, the 60 hours actually on the target, 10 hours of slewing around to get. To get onto the target. So a 70 hours data set, essentially. It is extraordinary because we can do so much with it. It turns out we can also, for the first time ever, measure the obliqueness of an exoplanet.
So we assume planets are normally spherical. They're not. They actually, they bouge out a little bit, you know, just kind of like love handles a little bit on the outside. And that's because of their spin. And so we could actually measure that for this exoplanet to very high confidence. If it has a Jupiter like ablateness, we could measure rings, we could, you know, find the moons, of course, we could actually measure the atmosphere of this planet. So this is a really special planet, a special data set, and we're hoping we'll see what comes out of the data, that it could really trigger a tsunami of new work in this field.
Well, we'll be watching to hear of the results in October. And thanks. Thank you very much.
Science, Technology, Education, Exoplanets, Astronomy, Astrophysics, World Science Festival
Comments ()