ENSPIRING.ai: Dark Matters - Have We Really Failed To Identify Most Of The Cosmos?
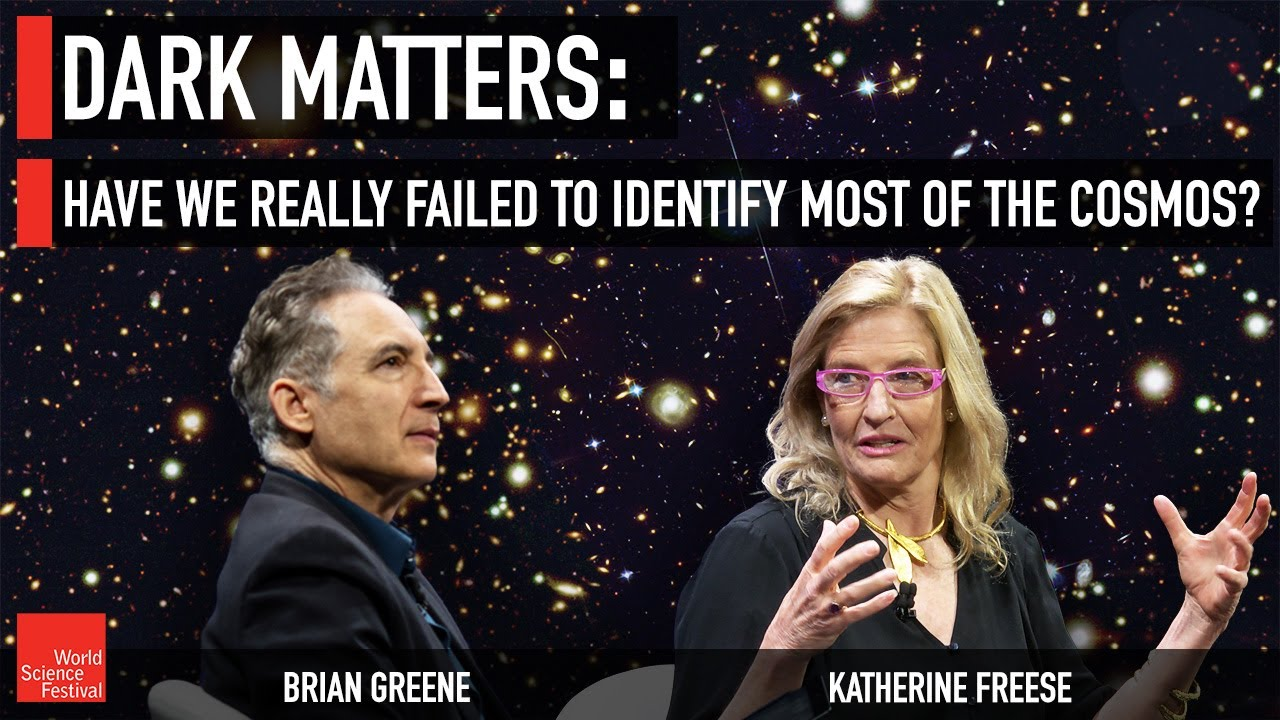
The video explores the profound concept of dark matter and dark energy, which constitute roughly 95% of the universe's mass-energy content. Although their presence is inferred through gravitational effects, neither has been directly observed or fully understood. The discussion highlights how historical figures like Fritz Zwicky and Vera Rubin contributed significantly to the understanding that galaxies contain much more matter than is visible, leading to the widespread acceptance of dark matter.
The episode features Katherine Freese, a noted physicist working to identify dark matter and dark energy. She discusses the challenges of studying these phenomena, the limitations of current models, and potential breakthroughs. The conversation touches on past theories that have been disproven, the Bullet Cluster's evidence for dark matter, and innovative concepts like "dark stars." Katherine also speculates on the existence of a "dark big bang" that might explain dark matter's origins, highlighting her research's intersection with gravitational wave studies.
Main takeaways from the video:
Please remember to turn on the CC button to view the subtitles.
Key Vocabularies and Common Phrases:
1. electromagnetic spectrum [ɪˌlɛktrəʊmæɡˈnɛtɪk ˈspɛktrəm] - (n.) - The range of wavelengths or frequencies over which electromagnetic radiation extends. - Synonyms: (radio waves, x-rays, gamma rays)
Air, of course, but yet more elusive examples are x rays and radio waves and infrared rays. These are elements of the electromagnetic spectrum that are outside the sensitivity of our eyes.
2. gravitational waves [ˌɡrævɪˈteɪʃənəl weɪvz] - (n.) - Ripples in spacetime caused by some of the most violent and energetic processes in the Universe. - Synonyms: (ripples, waves, undulations)
We have learned that we are also immersed in a sea of incredibly weak gravitational waves.
3. dark matter [dɑːrk ˈmætər] - (n.) - A form of matter that does not emit light or energy, detectable through its gravitational effects. - Synonyms: (invisible matter, non-luminous matter, unseen mass)
Evidence that we will review in this conversation shows that something on the order of 95% of the mass energy of the universe is carried by dark stuff, by dark matter, by dark energy.
4. theoretical physics [ˌθɪəˈrɛtɪkəl ˈfɪzɪks] - (n.) - A branch of physics that uses models and concepts to explain physical phenomena. - Synonyms: (conceptual physics, mathematical physics, speculative physics)
And our guest for this conversation is striving to better understand this dark side of reality, and therefore, it is a great pleasure to welcome my colleague Katherine Freese, who is the director of the Weinberg Institute for theoretical physics.
5. galaxies [ˈɡæləksiːz] - (n.) - Large systems of stars, interstellar gas, dust, and dark matter, bound together by gravity. - Synonyms: (stellar systems, star systems, cosmic assemblages)
They looked at galaxies and found evidence for dark matter in every single one.
6. gravitational lensing [ˌɡrævɪˈteɪʃənəl ˈlensɪŋ] - (n.) - A distribution of matter, such as a cluster of galaxies, that bends the light from a background object. - Synonyms: (light bending, light distortion, lensing effect)
So I can imagine someone saying, but if you can't see it, how do you know it's there? And that gives this idea of gravitational lensing
7. axions [ˈæksiˌɒnz] - (n.) - Hypothetical particles proposed as a component of dark matter. - Synonyms: (theoretical particles, conjectured particles, speculative entities)
axions, a great idea, also theoretically motivated there.
8. wimps [ˈwɪmps] - (n.) - Weakly Interacting Massive Particles, hypothetical particles that may constitute dark matter. - Synonyms: (hypothetical particles, conjectural particles, presumed entities)
And what I'd like to focus on are the two that are the best, theoretically motivated, and that would be the wimps and the axions
9. cosmic microwave background [ˈkɒzmɪk ˈmaɪkrəʊweɪv ˈbækˌɡraʊnd] - (n.) - Thermal radiation left over from the time of recombination in Big Bang cosmology. - Synonyms: (CMB, primordial radiation, remnant radiation)
Well, I like the idea of connecting the dark matter searches also with gravitational waves and also with cosmic microwave background experiments
10. supermassive black holes [ˈsjuːpəmæsɪv ˈblæk həʊlz] - (n.) - Enormous black holes found at the centre of most galaxies. - Synonyms: (colossal black holes, gigantic singularities, massive voids)
So, this is one of the better ways to make supermassive black holes
Dark Matters - Have We Really Failed To Identify Most Of The Cosmos?
We are surrounded, right, by physical substances that we know to be real, right. But which, to the naked eye, are invisible. Right. Air, of course, but yet more elusive examples are x rays and radio waves and infrared rays. These are elements of the electromagnetic spectrum that are outside the sensitivity of our eyes. And, of course, since I guess it was around 2015, we have learned that we are also immersed in a sea of incredibly weak gravitational waves. So here's the thing. When we look out into the cosmos, we should perhaps anticipate that at least some of what's there might also be invisible, not just to the naked eye, but perhaps also to our most refined equipment.
The surprise, then, is not that there is invisible stuff out there, stuff that for historical reasons, we don't call invisible, but rather we call dark. Rather, the surprise is how much dark stuff there is. Evidence that we will review in this conversation shows that something on the order of 95% of the mass energy of the universe is carried by dark stuff, by dark matter, by dark energy. And yet, here's the thing. This evidence, it's all indirect. We recognize the presence of dark matter and dark energy by their gravitational impact on their surroundings and on the universe as a whole. But no one has ever directly held or trapped or directly examined dark matter or dark energy up close and personal.
So here's what this means. For all the progress that we have made in understanding the universe. We are still in the dark regarding some 95% of what is out there. And our guest for this conversation is striving to better understand this dark side of reality. And therefore, it is a great pleasure to welcome to our stage my colleague and friend, Katherine Fries, who is the director of the Weinberg Institute for theoretical physics, and the Jeff and Gail Kadosky professor of physics at the University of Texas, Austin. Her path breaking work seeks the identity of the dark matter and dark energy and root, hopefully, to a testable understanding of the early universe.
Brian, so it is great to see you coming back for another conversation here at the World Science Festival. Thank you, Brian, for having me on. Absolutely. Jump right in to this question of dark matter, which has, you know, a long history. I guess it was at least as far back as the 1930s that people began to think about.
Now, the history that I know is, you know, people like we can bring up an image of Fritz Wicke, who maybe is the early to mid thirties, began to do observations and calculations that suggested to him that there was dark matter. You know, I'd always heard that he was the first to really bring up this idea of, well, he was swiss, so he called it dunkle materia for dark matter. Turns out in 1930, which was several years earlier, there was a swedish scientist, Knut Lundmark, who also realized that some things were moving too rapidly. How do you explain that? Because there must be some kind of dark matter. So he was there earlier, even.
And because you happen to have mentioned this earlier, we were able to put together an image of this individual who I have heard of, but I didn't know, perhaps had claim to the earliest prediction of dark matter. So that's a wonderful education for me, too. I learned about it during my time in Sweden as director of Nordita, the Nordic Institute for theoretical physics. So I heard about it from some swedish scientists, and then I heard about it again from people who study the history of dark matter in the United States. So you have corroborated this here in the United States? Absolutely. Yeah. Well, that's amazing.
Now, the person somewhat more modern who was vital to the subject is Vera Rubin. And with colleague Kent Ford, really began to make this subject compelling and quantitative. And so what observations were Vera Rubin and Ken Ford undertaking to come to this conclusion? Well, after the original stuff in the 1930s, there was a lot of debate, but it was really, in the seventies, the work of Ford and Rubin that nailed it, because they looked at galaxies and found evidence for dark matter in every single one. And that's when the scientific consensus really was, okay, this stuff, it's there. It's real.
So what they looked at in individual galaxies was if, let's say, here's the center of the galaxy and here's some gas or stars moving around the center. How fast are those stars moving? Well, that speed would be determined by the amount of mass that's interior to their orbits. And if you only look at the stellar light, then they should be moving, you know, tooling along. But woof, they're whizzing. They're moving really fast. There's some other mass in there speeding them up.
In fact, they're moving so fast that they should have been flung off. In fact, we have a nice little analogy that I think really captures that idea. This is a bicycle tire with an enormous amount of water on it, and as it spins, the water is flying off because there's not enough to hold it in. And that's sort of like these galaxies that you were making reference to. The stars should be flung off, but they're not. Yeah, yeah. I love the analogy with the bicycle wheel. Things can't. Something has to be keeping them in. If they're moving that fast. And the only thing that really survives a variety of other tests that we'll speak of is some kind of matter that we don't see. That's. Yeah, and that's. That's what it comes down to.
So when we look at galaxies, what fraction of the galaxy than would be dark matter according to this kind of reasoning? Well, the weird thing about dark matter is that although we don't see it, we sure see its influence. And, for example, in our own Milky Way, there's five times as much dark matter as there is ordinary matter. So most of the mass in galaxies is not the visible light. It's something dark, which is kind of strange because we spend a lot of time examining the stuff that we can see, but it's almost like, you know, looking under the lamppost for your keys. It's the only place that you have the capacity to do the analysis and the observations. But there's so much more.
Well, the other thing that's weird about it, of course, is that everything in our daily lives, our bodies and chairs and stars and planets, all made of atoms, we understand something about atoms, but all of that atomic matter, that only adds up to 5% of the universe. And the dark matter is made of something exotic. So not only doesn't it give off light, but it's just something really that we really don't understand yet.
And so we're going to talk about some of the candidates for the dark matter. But do you have an intuition that the dark side of reality is a whole nother set of insights, and perhaps even laws that differ from the laws that govern the stuff that gives off light or can reflect light? Or is it kind of more of the same? It just doesn't give off light. That's the only difference. Well, that, of course, is a. That's a really good question. Well, thank you.
So, we don't know. I mean, we know of the four fundamental forces of nature, and it's pretty clear that dark matter does feel gravity. The definition of mass is that it feels gravity. But then the question is, does it have any other interactions with ordinary matter and, well, electromagnetic interactions, the giving off of light? No. And strong interactions that hold our nuclei together? No. So weak interactions we'll get back to.
But so maybe, maybe in the worst of all possible worlds, sitting in a dark sector somewhere, talking to us only gravitationally, then how are we ever going to test it? Well, yeah, so I vote for the idea that it's not neutrons and protons, it's not ordinary atomic matter, but it's some new kind of particle, and we do have the ability to look for it and detect it. It's also good to nail the case as strongly as one possibly can. And this example of the bullet cluster, that's a good one, is, to me, when I saw that, that was sort of the moment.
I mean, look, my very first paper as a graduate student was on a dark matter candidate, because back in the 1980s, if you wanted to get started in research, your advisor would typically say, eh, just make up a dark matter candidate, work out the mathematics, and see what it might predict. So, no, wait a minute. Wait a minute. Vaguely. Vaguely. But when I saw the bullet cluster, that's when it became not abstract mathematics.
And so there are a series of images that we can walk through here, but can you just set up. So I gather there are these two galaxies that were crossing each other, and I'll show images that highlight the visible matter and the supposed dark matter. Can you set it up for us, though? Two clusters of galaxy. Two clusters of galaxies. So each cluster containing hundreds of galaxies, those collided with each other. And then you can see how different types of matter behave during and after those collisions. We get a snapshot right after the collision, which is absolutely beautiful.
So let me show this. So this red highlight, if I remember the color scheme correctly, is the visible matter that resulted from this collision. And the idea, I gather, would be, as these clusters pass through each other, the matter that interacts more strongly, the stuff that we can touch and feel, ordinary matter that will be kind of slowed down, almost like by a frictional wind from these clusters going by one another. But like you said, the dark matter is kind of standoffish, right. It doesn't interact very much. So if there is dark stuff, it should keep on traveling even though the ordinary stuff slows down.
Yeah. So that's gas that we see in x rays. And it is. Though you and I collide, we're not getting very far Right. And so it sits there in the middle and. Right. But then if we look at the gravitational influence of the other material out there, and that's what's highlighted in blue, we absolutely see evidence that stuff has gone beyond the visible material, and we know it's there because it's gravitationally influencing its environment.
And so if you overlay these two, you see these clusters as they go through. Well, there's definitely two different types of matter here. The one type, as you said, that's the ordinary matter that you see. It's shown in pink, and it gets stuck in these collisions. But then there's the other type of matter keeps going, and it's shown in blue. And these are two very different things, two types of dark matter.
So this blue stuff doesn't only not give off light, but it doesn't have the other types of interactions either. It's able to keep going. It's really clear evidence that there's two different things going on here, two different kinds of mass. And what role does an image like this play in your confidence? That, oh, yeah. Well, people had thought about alternatives to dark matter that would involve modifying Newton's laws. This was modified newtonian dynamics. And good luck creating this with those alternative models. I don't think anybody disagrees with the existence of dark matter anymore, because the alternative models just cannot give you this.
Well, there are people who do disagree. I have never seen them explain that in a convincing way. No. Okay, maybe. No. But even the people who are still holding out for Mond type of models, they agree that dark matter has to be there on large scales. They're saying Mond is a better description on galactic scales. But when you get really, really big, they're all putting dark matter in there now because you just can't get any of those other explanations to actually work here. No. And before we go on, though, I just want to make clear how it is that the blue is detected because you can't see it. So I can imagine someone saying, but if you can't see it, how do you know it's there? And that gives this idea of gravitational lensing. So you can give us a feel for that technique.
Yeah, this was predicted by Albert Einstein in 1915. And what basically what he said is that wherever there is mass, it's going to bend light. So although the dark matter doesn't give off light, if you have some distant, bright object, the dark matter will bend that light. And by studying how that the images that you see, because of that bending, you can figure out how much dark matter there is, where it is. Oh, this is a great image. So that is what we see. So starting with a background object, then these light paths got bent on the way to us. And in the end, you have a single star looks like you have multiple copies of it, and a single star looks. Here's another example of the multiple images where the lensing, the focal point is not by a glass lens. It's by the gravitational influence of this dark stuff.
And with that, we really are confident that that dark stuff, the blue regions, are actually out there. For instance, in the example that we're looking at a moment ago. So, with the case for dark matter being strong, what about potential dark matter candidates? Because, as you say, it's pretty tough to make everything work with protons and neutrons. You can't simply say it's collections of protons that somehow are not given off light. I mean, that kind of an explanation doesn't work. So what do you do then, if ordinary matter doesn't work?
Well, then people are trying all kinds of dark matter candidates. So the mass range that people are considering is enormous, all the way down to where they're barely massive enough to remain in the galaxy or all the way up to macroscopic objects. So people are trying all kinds of candidates, and some of the ones that we're showing here have been ruled out. They're not there. And so, systematically, we're going through a series of possibilities. And what I'd like to focus on are the two that are the best, theoretically motivated, and that would be the wimps and the axions. And the reason I say that is because they weren't invented just as dark matter candidates. That's critical. Yeah, that's critical. In grad school, they told you invent a candidate. Well, that's not how these arose. I agree.
Yeah. These were already there for other reasons. In particle theory, they solve other problems as well. In fact, those other problems, the solution to those other problems, automatically generated new dark matter candidates. They're just sitting there. That's really important. Yeah. And so why don't we. We can bring that back up. We can focus on the wimps for just a second.
That, to me, when I was a graduate student, I was being a little facetious in my description of what my advisor had told me. It was actually to try to use what are known as particles that come from super symmetric theories. And to me, that's a prototype of wimp. So WIMP, weakly interactive massive particles is the acronym. And so were you compelled by super symmetric dark matter candidates at some point? Yeah. Yeah. So supersymmetry solves some problems in the standard model of particle physics on the theoretical grounds. I mean, we don't know if it's true, but for every particle we know, there would be a partner. So you double the number of particles, but most of those would be heavy, and the ones that would remain would be perfect WiMP candidates.
And so, yes, that was a really powerful motivation. When you do the calculation of how many of these lightest of the supersymmetric particles, which would be the stable. Oh, yeah. This is how many of them should have stuck around since the big bang. And this is utterly mind blowing. It is. So I was talking about the four forces of nature, and I said, we'll get back to the we force because that is the one that these wimps would feel. And we can calculate in the early universe how many particles there are of every type in this hot thermal bath. And then as the universe cools, well, you have a lot of these wimps of their own antimatters, and we watch that annihilation process, and then eventually the universe expands to the point where these annihilations stop. And then you ask, okay, if that annihilation happened with the weak force, how many of these are left today? And the answer is right. To solve the dark matter problem. So you have these particles that are well motivated, theoretically, and they automatically solve the dark matter problem. Some people call this the wimp miracle, though I don't know if that's going too far, but yeah.
And so, certainly when I was a graduate student and for many years after that, the view was it was almost a foregone conclusion that the dark matter had to be one of these weakly interactive massive particles, one of these super symmetric particles. But then we look for them directly at, say, the Large hadron Collider or various dark matter detectors. What have we found? Yeah. No, well, so, you know, I like to say you can make them, shake them or break them. Those are the different ways to look for them. And so at the Latch Hadron Collider, you'd collide protons and make them and. Haven't seen anything yet. Frustrating. And then, as the other idea, I was one of the people that wrote the papers that got these direct detection experiments going. If there's all these particles flying around, well, if you have a nucleus, then the particle hits the nucleus, it'll deposit some energy in there.
You have to go deep underground to get away from competing signals. And people have built those detectors all over the world now, everywhere in Europe, in Asia, in the US, in Canada, even at the South Pole. And what have they found? Well, there's one experiment that claims. It continually claims to have a specimen that continually claims, and that's the italian dama experiment. Right. And it's the only one. Do you think it is anything? I don't know. I don't know. They're really. They will not release their data. They won't share their data to anyone. That's really weird. That's. But on the other hand, no one has an explanation for what they are seeing, so it still remains possible as a consequence, there are now four other experiments finally made of the same material, which is the only way that you could check it.
So you have to take data for some years. So we should be able to tell, but not yet. So a couple more years, five more years. But even so, you'd still have to address the question of the difficulty of not detecting these in any other detection. Why haven't the other experiments seen anything? That's a very interesting puzzle.
So that's. That's this natural candidate. Frustrating. That hasn't yet established itself and perhaps will. We don't know. What about this other axions that, that you mentioned? axions, a great idea, also theoretically motivated there. The theory of strong interactions that holds our nuclei together had a serious problem with it, and people found a way to solve that problem. And guess what? You automatically get a dark matter candidate. And these things are called axion like particles. And the experimental search is all over the map of what people are looking for. So this is, I think, a really important direction.
As you begin to think about the near future, what would it take for you to give up on the idea of dark matter as the right solution? Is there anything in the next few years that shake that I'm going to. There's two types of answers to this question. I mean, two subsets here. One is, are you talking about, am I going to give up on particle dark matter? And in which case, for example, I would take at this point, primordial black holes very seriously. So that's another way to go. Some of these other candidates are really interesting.
But then the bigger question of, do I give up on dark matter entirely as a concept for explaining all of these different pieces of evidence we saw. And my answer is no, I don't see any other way. I want to turn to some other fascinating work that's very related to everything that we've been speaking about so far, that you've been spearheading this idea of dark stars. Oh, yeah, my baby. So what are they? And then I think you actually even are going to show us potential observational data that. Yeah, so I'm excited about this one.
So what is a dark star? Well, the very first stars that form, and this would have been 200 million years after the big bang. As a reminder, the universe is 14 billion years old. So that was very early on, the very first light, the very first objects, they could have been very different from normal stars. Instead of being powered by fusion, they could have been powered by dark matter. Now, I have to make it really important to point out they're made of hydrogen and helium from the big bang. 99.99%. And the dark matter is a tiny, tiny piece of the total mass, but there's so much of it inside these early objects that it can provide a power source for the stars. It's more like a fuzzy cloud in which the dark matter can find itself. And we have a little animation just to give a feel for what that would be like.
So, tell us what we're seeing if we're going to go into this. We're going into the dark star. Yep. And at the center there, you have the dark, very high dark matter concentration with the dark matter particles annihilating with each other. And what that means is whatever they annihilate into gets stuck inside this star, so it interacts with the hydrogen and it can't escape. And what that means is you're dumping a heat source from the dark matter directly into the stars. A totally weird star, by the way. The radius, ten times the distance between the earth and the sun. Very strange. So 930 million mile? I don't know. Yeah.
Okay. And so, after a while, I gather that these dark matter annihilations will exhaust themselves. And so what would be the fate of one of these dark stars that form so early? What happens? Well, this is cool. They start out small about weighing about as much as the sun, but then they, because they're cool, they keep accumulating, accumulating mass, getting bigger and bigger, maybe as big as a million solar masses.
But once that dark matter source is gone, whomp collapsed the black holes. And that is a really good thing, because there is the big black hole problem. How do we explain giant, billion solar mass black holes? Ooh. Here we're making a black hole. And so this could be a signature of dark stars. Yeah, yeah, yeah, yeah. So, this is one of the better ways to make supermassive black holes. Maybe a million times the mass of the sun, and then they merge together to make a billion times the mass of the sun. And it's pretty hard to do that. Right.
There is a puzzle. I mean, most galaxies have a gigantic black hole in the center. Every galaxy has one. Yeah. And you have to explain where in the world this enormously massive black hole comes from. You suspect there are some observations that. Yep. Now, that's kind of cool, because you and I and our lot typically are doing theoretical things that don't always brush up against data that we can actually point to. So, can you take us through, I guess this is an image from the James Webb space telescope. And so what are we seeing here?
We wrote the original paper in 2007. And then in 2010, we heard from John Mather and John Gardner, John Mather, the Nobel laureate who started James Webb space telescope. And he said, oh, we could see those. Tell us what we will see. So we made predictions at that point. And so once James Webb turned on, we were waiting, can we get some candidates, please? And the James Webb is unique as far as how far back it can look in time, because the light from then was stretched to the infrared now, and that is a wavelength band that for the first time, we can see this far back in time. And sure enough, there are candidates in there, and that is one of them.
And so what do you think that is? And what about the measurements lead you to think that it might be a candidate? We looked in particular. What you need is spectra. In other words, how much light there is at different frequencies of emission. And at the time, this was a year ago, there were only five objects from the early universe that had spectra. And so we looked at those and of those, we realized that three of them are perfectly good candidates because one of the predictions we've made are, what are the spectra, what they should look like? And it matches.
So we don't know because one dark star is as bright as an entire early galaxy as stars. At this point, we don't know because the spectra are not good enough. If you only see hydrogen in helium, that's a dark star. If you see carbon, nitrogen, all that other stuff that comes in later generations of stars, then it's a galaxy. So what we need are better spectra and. But we will get them in the future because there we're talking about this lensing that magnet. It'll, again, you'll have a distant object that has a bunch of mass in front of it, which means you'll get a magnified version of that star and you'll get a better spectrum and then you'll know, aha, there it is. We see a particular helium line. It'll actually be a smoking gun. So if you see that, then you're done. You've got a dark star.
And so what level of confidence do you have in this right now? Is it just tentative or. You know, Brian, I'm never one to say I think it's this. I believe that. Da da da. I'm always rather conservative, and I'm going to say it could be a dark star, it could be an early galaxy, and time will tell. Yeah, I have to have it in front of the actual data in my face to believe it. Yeah. No, I think that's the rational way of going forward.
Now, in thinking about the subject, you've also spoken of sort of an augmented big bang, if you will. That sort of has a dark sector to it. The dark big bang. Yeah. So you can tell us what is that about? And, in fact, we have this little graphic that sort of lays out bit of the timeline of sort of the usual big bang and the dark big bang as well. So how should we interpret what we're seeing here?
Well, the usual big bang comes after a period of. After a period of inflation. Then the vacuum energy from the inflation converts into all of matter, including dark matter, regular matter. That's the standard picture of what is the big bang. But then we decided, we thought, well, wait a minute. How do we know that the dark matter is also created at the same time? And we decided to posit the question, let's have a second transition like inflation, and again, a dark vacuum energy that turns into dark matter and see how late, how far forward in time can we push that second big bang, the dark big bang, and still explain the universe that we see today?
And what do you find? Well, the answer is it's, you know, it's human scale. It's about a month. Now. That sounds short in human time. Huge. Right. Cosmological terms. In cosmological terms, that's enormous. So you. In the early universe. In the early universe, that's an enormous amount of time. Right. And so does that have observational consequences that you guys have worked out?
Yes. So we were looking, in particular, at a transition that happens with collisions of bubbles. So you were stuck in a high energy vacuum, and then you transit it. It's kind of like boiling water. And so you have bubbles of the true vacuum showing up. And when those bubbles collide, you get gravitational waves. And that is a major, major field these days. And in particular, there is an instrument called nanograph that is seeing gravitational waves.
And, yeah, this would be a perfect way to explain those. The traditional explanation is it's merging supermassive black holes. That could be, but the problem there is that it's hard to get the black holes to merge. They kind of get stuck. It's called the final parsec problem. But anyway, so we don't have that problem, but we have this speculation that we have these bubble collisions that are producing the gravitational waves.
And so if you were to compare where things are now to where things were, say, in the eighties. Right. Oh, boy. So it's a funny thing that the idea of dark matter has been around for this enormous stretch of time. I mean, do you think that things are radically improved in terms of our understanding now, or have we been just kind of chasing our tails for a while? No, don't put it that way. It's. It's been frustrating. I mean, if you'd asked me or anybody else in the eighties, we would have thought, oh, we're going to find this in the next decade and that. And then there were hints of detection, right? So there's also towards the galactic center, excess gamma rays, all kinds of hints of signal, and we're still sitting on those hints of signal.
So that is. That is new, I guess, but it's very frustrating. But you remain nevertheless optimistic that this is the right approach from everything that we've seen, and perhaps we will be able to detect something in a reasonable period of time. Well, I like the idea of connecting the dark matter searches also with gravitational waves and also with cosmic microwave background experiments. You can see what's going on a lot in the universe from this leftover light from the big Bang, and so there are signatures there that you can look for tying all these things together. I think that's the way to go. Hopefully, we will do it maybe in our ever shortening lifetime that we've got left on this planet. But thank you so much for illuminating us, Sadeena.
Science, Technology, Education, Dark Matter, Astronomy, Physics, World Science Festival
Comments ()