ENSPIRING.ai: ROBOFORMING - The Future of Metalworking? (I Had NO IDEA This Was Possible) - Smarter Every Day 290
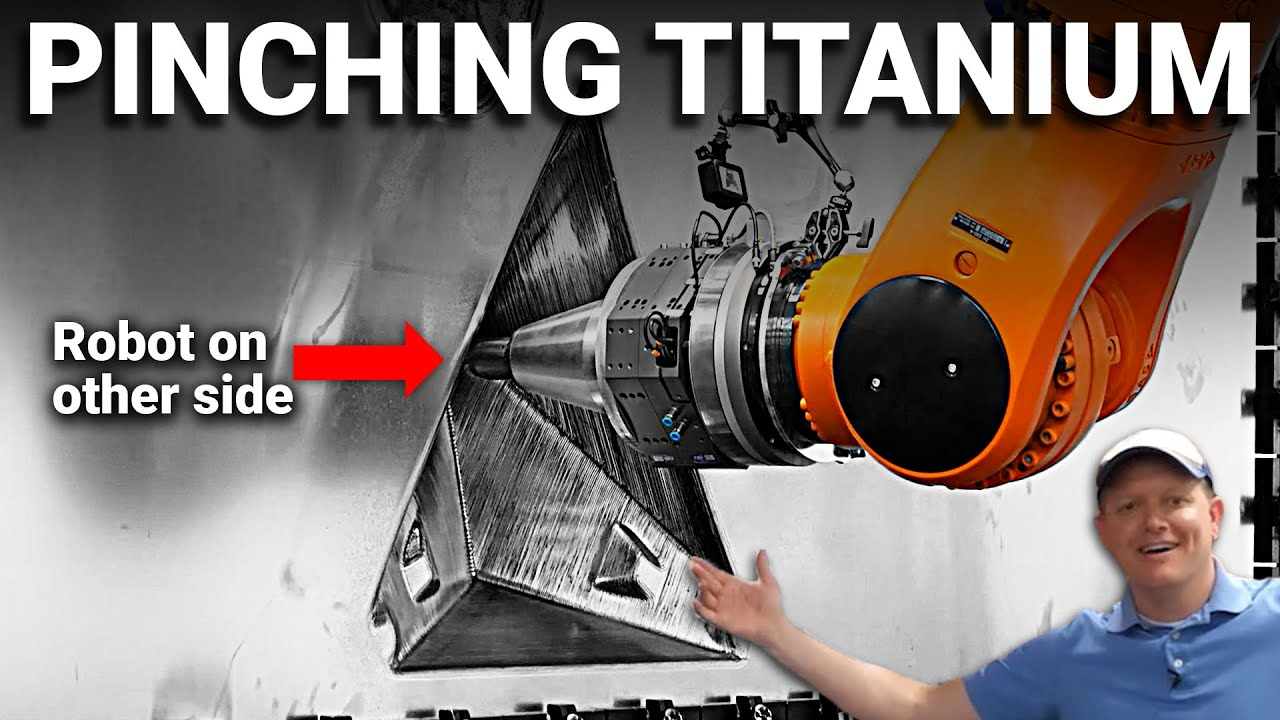
This video explores a fascinating new manufacturing method called incremental sheet forming, which is part of Destin's Smarter Every Day series on manufacturing. The technique allows for the rapid creation of metal parts through a process that is not as fast as traditional stamping but provides the flexibility to adjust and iterate designs quickly. The focus is on a company called Machina Labs in LA, which has developed a robotic approach to this process, branded as "roboforming."
The incremental sheet forming process is likened to the work of a potter molding clay, but with metal, using specialized tools called end effectors. This method permits the formation of complex and varied shapes without the need for molds, making it highly suitable for development and small-scale production. The video features insights from the Machina Labs team, who demonstrate how their system works and discuss the precision and control required to manage forces and deflections in metal forming.
Main takeaways from the video:
Please remember to turn on the CC button to view the subtitles.
Key Vocabularies and Common Phrases:
1. roboforming [ˈroʊ.boʊˌfɔːrmɪŋ] - (n.) - A robotic process of incremental sheet forming where metal parts are shaped without molds, offering flexibility and rapid iteration capabilities. - Synonyms: (robotic forming, automated shaping, metal crafting)
So let's go get Smarter Every day and learn about incremental sheet forming, or as this company calls it, roboforming.
2. effector [ɪˈfɛktər] - (n.) - A device or tool that moves or manipulates a material within a machine or robot, particularly in automated processes. - Synonyms: (tool, actuator, manipulator)
What do you call these tools? Call them end effectors, but, you know, forming styluses, but yeah, end effective forming end effectors.
3. kinematics [ˌkaɪnəˈmætɪks] - (n.) - The study of motion without regard to the forces causing it, often used in the context of machine and robot movement dynamics. - Synonyms: (motion study, dynamics, mechanics)
Getting two industrial robots to be very accurate in this envelope is one task that we have to do through a lot of kind of calibration and proper kinematics
4. deflection [dɪˈflɛkʃən] - (n.) - The bending or deviation from the original course, particularly in materials under the application of force. - Synonyms: (bending, deviation, displacement)
So one of them is correcting for its own deflection under load because they're not infinitely stiff
5. iteration [ˌɪtəˈreɪʃən] - (n.) - A repetitive process of refining or improving a design, system, or process. - Synonyms: (repetition, refinement, cycle)
Because you can start making the part, you know, two hours after your design is done with the mold, you have to go through iterations on the mold
6. datum [ˈdeɪtəm] - (n.) - A reference point, line, or surface used as a basis for measurement or calculations. - Synonyms: (reference point, benchmark, standard)
What they're talking about is wild because when you break metal, you normally have a rigid datum and you bend over a corner.
7. backlash [ˈbæklæʃ] - (n.) - The play between parts of a machine or gear train caused by gaps or mechanical imperfection. - Synonyms: (play, slack, gap)
It's a pretty complicated motion control problem, isn't it? backlash is also something you have to take into account with linear actuators, like this screw system here.
8. actuator [ˈæktʃuˌeɪtər] - (n.) - A device used to control a system or mechanism, often a part of robotic or automated machinery. - Synonyms: (control device, motor, module)
If you've got £5,000 of force that's in line with this first actuator here, there's not going to be any torque applied to it, right? But if you think about that second actuator, the offset between the force and the actuator will determine the amount of torque that that thing has to resist
9. restriking [rɪˈstraɪkɪŋ] - (n.) - The process of forming metal by multiple strikes or passes to achieve consistency in thickness and form. - Synonyms: (multistage forming, repeated striking, layered shaping)
So Ed took me to a whiteboard and he explained a complicated concept called restriking.
10. refractory [rɪˈfræktəri] - (adj.) - Describes materials resistant to heat and wear, typically used in high-temperature environments. - Synonyms: (heat-resistant, durable, hard-wearing)
Titanium inconel, we're talking about refractory alloys.
ROBOFORMING - The Future of Metalworking? (I Had NO IDEA This Was Possible) - Smarter Every Day 290
My brain's on fire. Hey, it's me, Destin. Welcome back to Smarter Every Day. We are right in the middle of a manufacturing Deep Dive series. And you may recall in a previous video, we went to a progressive metal stamping factory, and this place was incredible. They took coils of metal and they rolled them through these big presses with dies, and they would stamp out facts, thousands of parts. It's an incredible process. But one of the challenges to a process like this is it takes an incredible amount of time and skill to create these progressive stamping dies.
Today, we're gonna talk about a new process. Now, we're not gonna get the same level of accuracy or speed as we did in progressive metal stamping, but we are going to be able to iterate and create parts quickly, like change the part, which is an incredible asset when you're developing something or making a small number of parts. So today I'm super excited to continue the Smarter Everyday Deep Dive series into MEN manufacturing. And we're gonna learn about something called incremental sheet forming. The intricate details of how this process works blows my mind, and I've never seen this stuff on the Internet, and I think it's going to blow your mind as well.
So let's go get Smarter Every day and learn about incremental sheet forming, or as this company calls it, roboforming. So out in la, there's this company called Machina Labs, and after a little bit of back and forth on the intercom because people are rightly suspicious about a guy walking up with cameras to a secure facility, they eventually let me in. Oh, they opened it. Oh, nice. Okay, we're in. So this is Bobby. He's the one that reached out after seeing that I started a manufacturing series. He introduced me to a couple of his coworkers who helped me get signed in. And then we headed upstairs to the office portion of their lab factory where I met Ed and Babak, two of the founders of the company.
Ed is the CEO and has an interesting background in software, and Babak leads the technology partnerships and has a PhD in materials engineering. Both of these guys are astoundingly sharp. So my understanding is you guys have a way to create shaped metal by forming it by, I don't know how to say it. Touching it in a very special way is what I'll say. But let me just draw it right here. So you guys have a planar, a plane of metal, and then you bring two tools in and you can touch the metal like that. And depending on the position of this. What do you call these tools? Call them end effectors, but, you know, forming styluses, but yeah, end effective forming end effectors.
Okay, got it. So when these two end effectors come in and they put force right here, you can deform. You can deform the steel in there. And if you do it in a certain way, I'm assuming. I haven't got here yet, but I'm assuming the position of this versus this matters. Yes. Okay. And so you can do things that are really interesting. Yeah. So I think the best analogy that I can think of, you know, how a potter, you know, you have a clay on your turning table and the potter kind of pinches the clay with their finger and slowly deform it. That's what we're trying to do with sheet, but it's a very strong sheet and requires thousands of newtons to deform it.
Deformation with the pinch, it can be a shear, it can be stretching. There's a combination of different mechanisms happening until it gets you a part that you want so you have access to more interesting geometries. But the trade off is it takes a little more time to make the part. It takes a little bit more time to make the part. But if you take into account the fact that you had to make a mold to make the part, then in a lot of cases, up to even thousands of parts were faster. Really. Right. Because you can start making the part, you know, two hours after your design is done with the mold, you have to go through iterations on the mold. And usually the mold, the design of the mold is also slightly different than the design of the part. Right. Because the sheet has a spring back, you span bit, it comes off, it moves again. Right.
So your mold is usually different than your actual geometry. It's just not the negative of your geometry. Right. So by the time you're going through those iterations, you're spending hundreds of thousands of dollars. You get your part, you might be even faster just forming with us. But then once you're forming the part, obviously we're slightly restored than stamping. Stamping a few seconds to get your part. So this is great for development and low rate initial production. Yes. I think that's the first area that we're going after. But you were surprised where the break even point is. It's further down the chain. It's further than the chain. You. So Ed took us downstairs to see the robots.
But before you see it, I want you to think about this. This is not like these guys invented this overnight this is the result of decades of research by professors and scientists. And in fact, like, if you look at the old patent history, you can see patents from Japan, Europe, Finland, all over the place. People have been trying to figure this out, and we're just now getting to the point where they can crack the code on how to do it. Ford, Boeing, Nissan did some R and D work in this, but nothing really commercialized out of those efforts. So you're running Kukas? We run Kuka. At the moment, the robotic system is Kuka, but we also can use fanics. So you can see on the other side. Those are Fanuc robots. Yep. We are robot agnostic.
Those are fan. Yeah. We pretty much basically build the whole control system for the robots from scratch. So it's not dependent on any features that the Kuka has. It's like a dance. Oh, that's amazing. It's different than I thought. It's actually pushing. Sorry, I'm being rude. I'm destined. Mark. What's up, man? Nice to meet you. How are you doing, man? Can I put a mic on you? Are you cool with that? Sure. Can you show me what's happening over here? Yeah. So this is incremental forming. Yep. Incremental metal forming. That's right. Yep. Two millimeter aluminum sheet, and there's one robot on each side, and they're sort of pinching, and it's a lot like a potter's wheel, sort of like spinning like that.
But here the tips are moving instead of the work piece. And it doesn't have to be round. Right. You can sort of push any shape you want. And so layer by layer, we're sort of stretching and pinching and pulling whatever shape I want here out of this metal. So. So the triangle here is coming out of plane. That's right. Which means this one over here. Are there any optical lockouts I need to be aware of? Nope. You can walk right up to it. Can I walk here? Yep. All right, thanks. Just try not to get between the robot and the sheet. You should be good. Yes, sir. So this one is pushing. That's right. So this rope, do you have a force gauge on this end effector? Yeah. There's load cells on both robots, so you can feel exactly what they're doing here.
You can see over here what's going on. This is the forces that both robots are feeling right now. Okay, so which. Can you tell me what colors are what? Yeah, there's the Z component, the in plane component, and then green is just the total. It's pretty Easy to understand the forces pushing into the plate, but the in plane forces are the side to side forces as the thing moves around. And so there's robot number one, here is the one that's pushing, and then robot number two here is the support that's on the other side. What you could do is you could do this process with just one robot and you could just sort of poke in metal and basically do a bunch of work by stretching.
But the boundary conditions like, then you load the whole thing up in tension, right? So what we're doing by having a support robot on the other side is you pinch and so you localize all the force just between the tips, which sort of reduces tension on the sheet and makes it so you can form a lot more accurately. That's amazing. Okay. What Mark just said was crazy and I have a little visualization here to try to help me understand it. This is just fabric on a sewing frame. Check this out. If you push against this thing, it's going to tent out, right? Like this is going to deform. Obviously this doesn't behave like the metal does, but it's going to deform out there.
Now when people work with metal, they use something called breaking in order to locally deform the metal past its yield strength. Basically, they make a permanent deformation. What they're having to do in this case is because they're poking on one side. If you were to push so hard that you deform it, it'd like tent out. It'd be really weird. Right. So they're locally doing it by putting two end defect right next to each other and just yielding it right there in that little bitty spot. That's really hard to do when you have forces on both sides and everything starts to move. So what they're talking about is wild because when you break metal, you normally have a rigid datum and you bend over a corner. They don't have a rigid datum. They're moving things around, which makes this complicated.
So one is pushing. So I would expect a positive force or a compressive force on that one and this one. Do you. If it was just holding in one position, would you just hold the robot here until it feels forced from the other side so that you know that you are actually pinching. We get pretty fancy about it. We're basically. Should I talk about all this stuff? Am I allowed to do this? Should I let him do it since it's ultimately his authority? No, no, no. I mean, I think Mark, Mark knows. Yes, go ahead. No, continue yeah, really, there's control systems on both of these robots where we can sort of plan their nominal trajectory that just sort of is the theoretical what they should be doing. They also like, they feel the forces, and we use that to update to make corrections to the trajectory base. Continuous feedback. Yeah.
So one of them is correcting for its own deflection under load because they're not infinitely stiff. The other one is trying to hit a target pinch force that we set. In super simple terms, the first robot is supposed to get to a certain point in space, and the second robot is supposed to push at a certain force required to bend the metal. That's interesting. But the problem is, once you start pushing at those levels of force, the robot itself starts to bend. So you have to accommodate for that. You have two robots on a pretty large envelope. Right. So the envelope is 12 foot by 5 foot. Getting two industrial robots to be very accurate in this envelope is one task that we have to do through a lot of kind of calibration and proper kinematics.
But then the moment they start touching sheet, the sheet will resist them. So. And these robots are pretty noodle. You can kind of imagine every joint slightly deflects. Right. So there are controls and mechanisms that basically compensate for that deflection. So that robot stays accurate under dynamic load. Right. Sometimes, depending on what sheet we are forming, it can be like 20,000 Newtons of force that you're applying. It's like as high as the weight of a truck on a very small end effector on the forming side. Right. So being able to. And under those loads, robots sometimes deflect six, seven millimeters. Right. And you want to pinch a sheet that's only 2 millimeters or half a millimeter. So we have to adjust for that. So there's a control loop that uses the force data, some of the other data, to constantly figure out how it can stay accurate and pitch the sheet the right way.
You just blew my mind a little bit. There's a lot of math here. So is there a robot around this corner? There's a robot, but it's not running at the moment. But can we. Okay, so if I understand what you just said, like, there's many ways to arrive at this point with this linkage. Yes. Okay. And if I arrive at this point and apply £5,000 in that direction. Right. I'm going to get a certain radial deflection here. Yes, I'm going to get a certain radial deflection here. You have to also determine how you get there in order to Anticipate the deflection. Yes. When you have a robot like this with a lot of different joints, you can be in one location, but you can get there many different ways, right? It's kind of funny, but you see what I'm trying to say.
Think about it like this. If you've got £5,000 of force that's in line with this first actuator here, there's not going to be any torque applied to it, right? But if you think about that second actuator, the offset between the force and the actuator will determine the amount of torque that that thing has to resist. And the same thing applies to that third actuator down here. And all of those forces eventually get transmitted down to the base of the robot. If you think about how these actuators work, it's made up of motors and gears, right? And if you were to zoom in, you would find that these gear trains are imperfect. If you have a driving gear here, if it were to stop and reverse and go the other direction, you would find that there's play between the gear teeth, and that causes something called backlash. Watch the robot draw this shape. Now think about how the motors change direction at different moments along the polygon.
Now think about the loading that's happening in the robot arm as it changes directions. It's a pretty complicated motion control problem, isn't it? backlash is also something you have to take into account with linear actuators, like this screw system here that's moving this big robot back and forth. When you move one way and then the other, you've got to take into account backlash there as well. Okay? So not only do we have issues with the actuators themselves, we also have issues with the arms themselves. Like, think about this. If you've ever been on a ladder and you've tried to, like, drill something in really high like this, and your arm's all weak because of where you're at. But if you're, like, up here, and you really put your power behind it and you line everything up like, you don't deflect as much, and you can get more strength out of it, all these things factor in as well.
So not only do you have the actuator movements, you have the rigidity of the arm itself. There's two types of accuracy. Like, get the robot to one point, but then also accurately move from one point to another while the forces are changing on the robot. So with the load now there's an extra, like, okay, the joint, a one not only need to be at 60 degrees, it needs to be at 60.1 or 60.2 degrees, depending if at the end the vector is facing, facing like a 5,000 pound force. Right. And including that into the kinematic calculation, that's hard. It's fun. It's a fun thing that our robotic team is working on.
So if this is at 0 degrees and I'm pushing into that, are there many different ways to set up the robot to get there, or is there only one way it can be set up? So because we have seven axes, there is probably. There's unlimited ways to get to that point with different poses. Right. So what do you do? Do you pick a few things and say, this always has to be here, this always has to be here? No. So there's so many cool things. You can just choose randomly choose. Or you can optimize, for example, for stiffness in certain direction that your force is going to be. So you say, okay, move the robot and the rail so the joints are in the most stiffest combination to apply the most amount of force without deflection. So there's like you have a function to optimize at that point. Right. And we can kind of partner your kinematics. You can figure out what exactly you want to do.
You're wicked smart, dude. No, I'm not. Yeah. This episode of Smarter Every Day is sponsored by NordVPN, which is a virtual private network that you can to mask your IP address online. You can get a ridiculous deal right now with Nord if you go to NordVPN.com smarter and the reason you don't see it on this piece of aluminum right here is because we're about to manufacture that. While I tell you more about NordVPN. While I start by getting this block down to size, I want you to know that the way I use NordVPN is to mask what I'm searching on the Internet. I don't particularly want people looking over my shoulder when I'm trying to learn about things I don't know a lot about. Sometimes those are political topics. Maybe they have something to do with health things. I'm wholeheartedly endorsing you using a virtual private network to maintain your privacy online.
NordVPN is super easy to use. You can even connect to the Internet from different places in the world. There's over 5,400 servers you can choose from in 60 countries. The desktop app has this thing called threat protection. This protects from malicious sites, downloads, trackers and malicious ads. One account gives you protected access to the Internet for six different devices. So you can use it on all the things. All right. You can see the URL is starting to come to life here. Nordvpn.com smarter get a massive discount on the two year plan specifically and you get four months free. Go check that out, see what you think. It's risk free because NORD gives you a 30 day money back guarantee. By the way, NordVPN has a no logs policy. They don't collect, track or share your private data. All right, so here it is. If you want to try this and support Smarter every day by supporting the sponsor, you can go to nordvpn.com/smarter like you see on the block of aluminum here. Thanks for sticking around for that build and thank you for considering supporting the sponsor. Because you're smart, you know what's going on here and I'm grateful that you would consider supporting.
Okay, that's just all the robot. Now we have to think about the metal itself. If we have a piece of metal and we push on it, it's going to deflect, right? If we hold it at two locations and we push on it, it's going to deflect in the middle, right? What happens if we're holding it here and here and we have to push on it down here? Like you're not in the middle, you're way over here. You're going to get a different deflection curve all along the way. Let's go back to my little funny piece of fabric here, right? Think about that. It's one thing to do it for a beam. That's what's called an indeterminate deflection beam. Civil engineers are really good at that. Think about a plane. It's way different. Like planar deformation is hard.
So if I were to push up in the middle of this thing, I were to get a deflection right there in the middle, right? It's going to be different if I'm over here at the edge. Because you see, the angle is different towards the edge than it is towards the middle. It's kind of hard to see this. But this is also something that has to be taken into account when you're doing all these calculations. What do you call it when, if I were to take a sheet and I were to touch in the middle, I would get more deflection than the edge. Is that edge effects? Is that what that's called? I think we call it like boundary condition effects. That's what you guys call it. Yeah, but you're right.
Boundary condition affects the accuracy of the parts that you get. So we have a whole software stack that tries to account for that. So you can imagine you form a part, you can then scan it. Actually the same robot scans it afterwards to do quality, to figure out. Yes. Exact quality, or should just figure out what it formed to update the model. And then it will be like, okay, I formed this. These are the areas that were off. That means maybe I should have pushed more in some areas and pushed less in some others. And you can manually iterate on that, keep generating data, and then later create a model that would tell you, okay, in order to form this geometry in this location in the sheets, you need to actually form a completely different part. And in the end you're gonna actually get the right part.
With all these things you talked about, you might overshoot here, undershoot here. Just like injection molding or all these processes have that. It's actually rolling, isn't it? Yes. So this specific one does. There are other ones that we have that rolled in different ways. Like a ballpoint pen. Yes, exactly. There's a sphere tip, There's a flat tip. Depending what kind of effect you want to get out of the part, you might want to use different energy, different radii as well. If it's a sharp crease. Different. Okay, so that detects the smallest feature that you can do. Like they've done like a quarter, quarter an inch diameter or some very fine detail features. I think These are what, 3/4? 3/4, 3/4 inch diameter.
Yeah. So this one is just. I can't predict where it's going. Mark, you have a complicated program. So. Yeah, it's rolling along the pointing axis, I'll say. And you can see at the corners where it sort of changes wall angle, where the tip sort of goes in and out at the corners. It changes. Yeah. Like right here. It's sort of at the bottom of the part. You'll see it comes out now and then it'll go back in. Oh, so I see. So we're moving in the Z. Yeah. Like this wall is steeper than this wall, and now it gets steeper again. So the tip sort of goes in and out there to basically keep the tips perpendicular to the surface that you're forming. Ah, I see.
So even though it looks like it's a 2D operation on any one slice, it's actually a 3D operation the whole way around. They're planar slices, but the tips sort of move like this, sort of depending on the wall angle that you're forming. Oh, really? And we have some fancy non planar slices too, if we want to talk about. I'll show you some of the parts there that are non planar. So like you're forming on a curved section. Yeah. So now you're not starting from a flat sheet, you're forming from a section that's already have forming in it. Now your robot is actually going into a non planar surface to deform and make another feature out of it. Oh, that's awesome.
So this is what's going to happen. Yeah, we're doing the face and then this triangle is the one that's just started over there. Right now this whole thing is about 90 minutes. Then it'll do this hexagon part. This one's actually a spiral rather than layers. So there's no, it's a 1 millimeter pitch, but it's just a spiral the whole way. Yeah. And then all of these are actually chained together as a single operation. So the robots will do one and they'll immediately go over and start the next one without slowing down. That's interesting because when I looked at it on the Internet, I thought you were going to do one plane and then just move in the z axis and do more and more planes.
But you don't have to do that. We can do whatever we can imagine. Like right here there's a bit of a seam if you look really closely where we transition. But if I do a spiral, then you won't find that on this part you transition, meaning you move up. Like this one is just planar slices. So you sort of do one loop and then you step in and you do the next loop. Oh, I see. The other one there is pathed as a spiral. So it's just a continuous sort of going a little bit deeper. Is it also faster? I guess a little bit. Because you don't have to turn around. So yeah, that one, it just kind of goes the whole way. Can you tell me about the end effector here? Yeah, so earlier version, right? Yeah.
So can I stand here? Yeah, of course. So the goal is, you know, we really, what we want to do is even though we are doing sheet forming, really our long term goal is to build what we call like a robotic craftsman. Like a system that works like a craftsman. Like, you know, you can pick up a forming tool, form it, drop the forming tool, pick up a scanner, scan it, drop the scanner, pick up a trimming tool. You can see there's a spindle there. Is that a. Is that an er, 20 something. Yeah, yeah. So the idea is like, yeah, you can easily change it. So you can see a tool changer here that can drop a tool, pick up another tool and just move on to the next operation. Right. And that's kind of the. We're imagining these robots, like, really working like a craftsman that can pick up tools and do different things.
Okay. So you're not pushing like, oh, you're applying electrical contacts. And then you have the mill here. Yeah. So again, once you pick up, it automatically connects through the electrical contacts. And now it's. The robot now has ending, milling, end effector. And they can drop this, pick up the forming end effector, go back to forming. So you have indexing pins to align it, and you also align on the plane so you have total indexing. And then what's going on here? So those are actually the bearings that through air pressure, they lock into the tool. Okay. So once it pick up the slave, this thing basically through air pressure, come out and then lock into the tool so the tool will not fall. Oh, that's amazing. And what is this? You told me, but I forgot.
So there are different connects. So these are pneumatic connections and these are electrical connections. Got it. Right now we do mostly forming and trimming. So we do mechanical trimming. So you can see that tool is in a spindle that allows us to cut the parts after we form them. And then you have different versions of the forming and the package. So that one doesn't have the final end effector that goes into it. Okay. It does the forming. The one next to it, it does. As you can see that the end effector is right in there. So your tools, are they carbide or is that too brittle? So we tried a bunch of material. Right now most of our tools are the base of it is carbide, but then on top of it we have a coating.
That coating is slightly more complicated. We have tried a bunch of different things. That's proprietary. Yes. You can base. That's the coding that basically allows us to do many parts for many parts without basically destroying the part or destroying the tool. It's a pretty simple tool, isn't it? Yes. So that the end effector is pretty simple. This is one of our designs. We have multiple designs. This is one of the design that we're working at the moment. Then the coding getting to that. That's the secret sauce. It took a while. It took a while, like 7pm running to UPS get this out. You know, even though that's a secret sauce, it really Also comes down to the software pieces. For example, if you say get the robots and run them in, just, you know, get the geometry, slice it in water lines and start forming it. The final part is going to be inches off of what you actually want, really.
So you have to really account for a lot of different things. We talked a little bit about deflection of the sheet spring back of the geometry itself, and then the fact that it can also tear. So if you don't have put right amount of compression force between the tips, you might tear the sheet. Right. So we get to the fracture limit and we tear the sheet. So combination. All those things are accounted in our software to get you a part that's going to be, you know, very close to the, to your final geometry and not like inches off. So that's I think, where the real see secret sauces, the software piece. So when you get done with this thing, you're going to have a fully formed sheet. This is going to be a hood for a truck. It'll be done.
How do you get it out of that? Do you like jigsaw it out or. So the same robot, after it's forming, it scans it and then maps the scan to the trimming path. So it aligns the trimming path to the scan. The best way picks up a trimming end effector and trims apart up. So you leave few tabs around the part and then technicians come in and cut the few tabs and take it out. But we want to make sure the robot at least does the accurate cutting so that the part before you remove it, before you move it, and then you leave few tabs in and then you cut those tabs and clean those tabs out. So if you had titanium like back in the day when they were building the SR71, they didn't know how to work titanium. I saw the glimmer in your eye.
To this day, I don't think they can easily form that can easily work titanium. Really. I think it's a tough, just a tough ally to work. I mean, we figured out how to machine it. But still forming it is very tough unless you elevate temperatures. And room temperature is really tough to form titanium. But in theory, could you use this technology to form it and then go in and cut the rivet holes? Yes. And are you very excited about that? I think that's that those are the areas that we are the most excited, like enabling alloys that were just impossible before. You know, I think for example, for Hypersonic, you said SR71, but all the hypersonic applications as the focus of attention a lot now with some of our customers, they have a very hard time forming skin of these aircrafts out of high temperature alloys.
Titanium inconel, we're talking about refractory alloys. Is this titanium? This is titanium. That's already cut out. The part from the customer cut out. But you can see the rest of the sheet. It's actually pretty thin sheet, super strong. This is all not part of the part. But this was kind of like the form. I don't know what the word is. We create enough stiffness around the part that as we are forming it, the part is not buckling. Got it. Right. Okay. So because I'm pushing here. Okay. Wow. That makes sense. Because if I'm pushing right here, if I'm so steeper angles give more rigid parts. Yes.
And the deeper it is, it becomes rigid and the deeper it gets. Also you are more rigid because you have to overcome a little bit of elastic deformation before you get to plastic. Yeah. So like, if you want to do a very shallow part, it just doesn't work. You're just gonna. You're just gonna push the part in and out. Once you go further deep, then you create more rigidity. You can easily overcome the initial elasticity and get to plastic regime faster. Right. My brain's on fire. The rigidity of this support skirt around the part is key. And a keen observer will notice in a lot of these parts laying around the shop, it's not just a flat crease with the back plane, it's actually a wavy gusset because that's a more rigid structure.
So if I'm looking at this sheet right here is the material the thickest it will ever be right here in the plane. And anytime I work, I'm having to move material. So it's going to be thinner. Yes. Here. Is that true? Yes. So I think the good law you want to apply is that kind of conservation of volume. Right. So as you're going on a higher wall angle, the cosine of your wall angle or sine of your draft angle times the original thickness roughly gives you the thickness you got to get in the part. Ed took me to a whiteboard and he explained a complicated concept called restriking. The quickest way to explain restriking is if I want to make a hemisphere in one shot. It'll make the sidewalls right around the outside diameter too thin.
But if you do it in two shots, first you make cone, and you push that cone out, then you'll have more material so you'll have more thickness on the outside of the hemisphere if you want to learn more about how this works. And a ton of different things that we didn't talk about in this video. I've got a lot of that stuff on this second channel video where we just let the conversation play out and you can get right into the weeds with us and understand all these really cool concepts. It's fascinating.
Say that again. That was huge. You can form parts faster than you could simulate it on the computer, right? That is such a big deal. Yes. A huge thanks to the folks at Machina Labs for showing us this stuff. Incremental sheet forming, or as they call it, roboforming. It's a really cool thing and I'm grateful that they helped us continue the Smarter Everyday Manufacturing series. Speaking of which, if you are interested in seeing the next videos in the manufacturing series, I have an email list. I'll leave a link in the video description or smartereveryday.com There's a link to the email list there. It's really simple. I don't spam you, I promise.
Finally, I want to say thank you to the patrons of Smarter Every Day. This is such a fun thing I get to do. It's a lot of work and the patrons make it possible. This community of people is the driving force behind me hitting schedules and like actually having the resources to get it done. I'm so grateful for everybody that contributes to Smarter Every Day on Patreon. If you would like to consider that, I'd be grateful. You can check that out@patreon.com absolutely no pressure here, but it's a really cool thing and we've got a good vibe going over@patreon.com smartereveryday if you'd like to check that out. I'd be excited about that.
Anyway, that's it. I hope you enjoyed this, the next video in the Manufacturing series. And if you want to learn more, there's more information over on the second channel in the long video. That's it. I'm destin you're getting smarter every day. Have a good one. Bye.
Innovation, Technology, Science, Incremental Sheet Forming, Robotics, Manufacturing, Smartereveryday
Comments ()